What type of Carbon is best to Capture?
“Carbon isn’t a difficult element to spot in your daily life. For instance, if you’ve used a pencil, you’ve seen carbon in its graphite form. Similarly, the charcoal briquettes on your barbeque are made out of carbon, and even the diamonds in a ring or necklace are a form of carbon (in this case, one that has been exposed to high temperature and pressure). What you may not realize, though, is that about 18% of your body (by weight) is also made of carbon. In fact, carbon atoms make up the backbone of many important molecules in your body, including proteins, DNA, RNA, sugars, and fats.
These complex biological molecules are often called macromolecules; they’re also classified as organic molecules, which simply means that they contain carbon atoms. (Notably, there are a few exceptions to this rule. For example, carbon dioxide CO2 and carbon monoxide CO contain carbon, but generally aren’t considered to be organic.)” – Carbon and hydrocarbons | Khan Academy
The carbon capture that benefits the earth, environment, and humans the most is to capture both CO and CO2, but they both have several options in attaining that goal. The methodology contained herein focuses on the capture of Carbon Dioxide CO2 and storing it in soils.
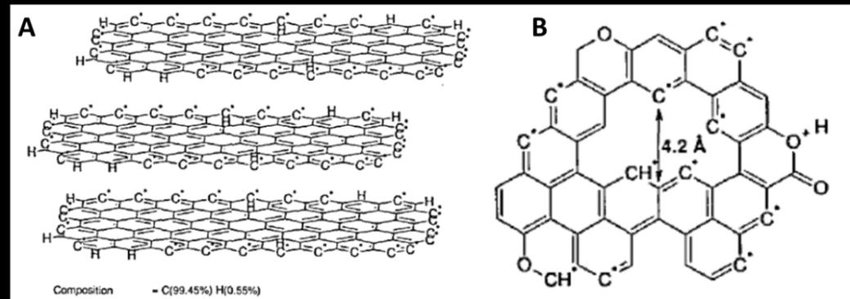
Carbon Sequestration Explained
“Carbon sequestration can mean capturing the carbon dioxide (CO2) produced from new and old coal-powered power plants and large industrial sources before it is released in the atmosphere. Once captured, the CO2 is put into long term storage either by storing it in carbon sinks (such as oceans, forests or soils) or underground injection and geologic sequestration into deep underground rock formations.
“Developing technologies to reduce the rate of increase of atmospheric concentration of carbon dioxide (CO2) from annual emissions of 8.6 Pg C yr–1from energy, process industry, land-use conversion and soil cultivation is an important issue of the twenty-first century. Of the three options of reducing the global energy use, developing low or no-carbon fuel and sequestering emissions, this manuscript describes processes for carbon (CO2) sequestration and discusses abiotic and biotic technologies. Carbon sequestration implies transfer of atmospheric CO2 into other long-lived global pools including oceanic, pedologic, biotic and geological strata to reduce the net rate of increase in atmospheric CO2. Engineering techniques of CO2 injection in deep ocean, geological strata, old coal mines and oil wells, and saline aquifers along with mineral carbonation of CO2 constitute abiotic techniques. These techniques have a large potential of thousands of Pg, are expensive, have leakagerisks and may be available for routine use by 2025 and beyond. In comparison, biotic techniques are natural and cost-effective processes, have numerous ancillary benefits, are immediately applicable but have finite sink capacity. Biotic and abiotic C sequestration options have specific nitches, are complementary, and have potential to mitigate the climate change risks.” Carbon sequestration by Rattan LalA forest is considered to be a carbon sink if the trees in it absorb more carbon from the atmosphere than it releases. Carbon dioxide is a vital gas. It is necessary for photosynthesis. Carbon is absorbed from the atmosphere through photosynthesis.
During photosynthesis, trees and plants “sequester,” or absorb, carbon from the atmosphere in the form of CO2, and turn water and carbon dioxide into oxygen and sugar called glucose. Trees take in carbon dioxide from the air and store it as carbon in forest biomass, that is, trunks, branches, roots and leaves, in dead organic matter like litter and dead wood and in soils. This process of carbon absorption and deposition is known as carbon sequestration.” – Rinkesh Kukreja Conserve Energy Future
Types of Carbon Sequestration
1. Biological Carbon Sequestration
This, roughly, is the storage of carbon dioxide in vegetation like grasslands and forests, as well as in soils and oceans.
In soils: carbon can be sequestered in soil by plants through photosynthesis. As such, agroecosystems degrade and deplete the soil organic carbon levels. Luckily, soil can also store carbon as carbonates, created over thousands of years when carbon dioxide dissolves in water and percolates the soil. The carbonates are inorganic and can store carbon for tens of thousands of years while soil organic matter stores carbon for a few decades.
Carbon is the main component of the organic matter that makes fertile agricultural soil. It also helps the soil retain water. Plants are the primary way that CO2 is transferred to soil. Not all of the CO2 that plants suck up for photosynthesis is needed for food. The excess goes down through their roots and feeds organisms that live in the soil. Carbon from the roots and leaves of dying plants is also captured in soil.
2. Geological Carbon Sequestration
This is where carbon dioxide is stored in underground geologic formations, such as in rocks. Industrial sources of carbon dioxide such as steel or cement production companies or energy-related sources like power plants or natural gas processing facilities will release their carbon dioxide, which is then injected into porous rocks for long-term storage. Such carbon capture and storage allows the use of fossil fuels until a substitute energy source is introduced on a large scale
3. Technological Carbon Sequestration
This is a relatively new way of capturing and storing carbon dioxide and continues to be explored by scientists. The method uses innovative technologies, which means scientists are also looking into more ways of using carbon dioxide as a resource rather than removing it from the atmosphere and directing it elsewhere.
- Graphene production: technology is being used to produce graphene from carbon dioxide as its raw material. Graphene is a technological material, used to create screens for smartphones and other technological devices. Its production is limited to specific industries but if carbon can be used to make more of the product, it might be a viable resource and an effective solution in reducing carbon’s emissions from the atmosphere.
- Engineered molecules: scientists are engineering molecules that can take new shapes by creating new compounds capable of singling out and capturing carbon dioxide from the air. These engineered molecules act as filters and only attract the element they are engineered to seek.
- Direct air capture (DAC): this is a means of capturing carbon dioxide from the air using advanced technology plants. The plants would seek to capture carbon dioxide from the air as the artificial ones do. It is an effective technological method of sequestrating carbon but it has its challenges. The project is energy-intensive and is also expensive to implement on a mass scale. It is estimated that between $500 and $800 is required for every ton of carbon removed.
4. Industrial Carbon Sequestration
This is not a widely renowned method, but it can be used in some industries. They capture the carbon in three ways from a power plant, pre-combustion, post-combustion and oxyfuel
- Pre-combustion: the carbon is captured in power plants before the fuel is burned. The aim is to remove the carbon from coal before it is burned. The coal is reacted with oxygen to produce synthesis gas, a mixture of carbon monoxide and hydrogen gases. The hydrogen is removed and either burned directly as fuel or compressed and stored in fuel-cell cars. Water is then added to the carbon monoxide to make carbon dioxide which is then stored and the extra hydrogen is stored with the hydrogen previously removed
- Post-combustion: here, carbon is removed from a power station’s output after the fuel has been burned. This means waste gases are captured and scrubbed clean of their carbon dioxide before they travel up smokestacks. This is achieved by passing the gases through ammonia, which is then blasted clean with steam, releasing carbon dioxide for storage.
- Oxyfuel or oxy-combustion: the point is to burn fuel in more oxygen and store all the gases produced as a result. Instead of laboriously separating the carbon dioxide from other waste gases, the process traps the entire output from the smokestacks and stores it all. Pure oxygen is blown into the furnaces to purify the exhaust, so the fuel burns completely, producing relatively pure steam and carbon dioxide gas. Once the steam is removed by cooling and condensation, making it into water, the carbon dioxide can be safely stored.
What can the average farmer do to increase the carbon capture ability of their land, and does that effort have any other benefits other than sequestration?
- Adding bio-char to any soil type can improve the soil’s mycology, water retention, erosion control, and organic life concentrations, especially when the charcoal is soaked in heavy fungal teas and microbial mixtures prior to application to the soil.
- Adding 1 ton of BioChar to the average field is suggested.
Only ⅓ of that is required if the char is activated. - Activating BioChar requires some soaking of the fresh char in microbial tea, which also has mycology in it. We take Gro-Kashi and make tea with EM1 product from TerraGanix that then has RootWise Biodynamic added which will need to mix for at least 30 minutes prior to soaking the char.
- For small batches of inoculation and soaking, we have put the soaking char in a vacuum chamber at 50 PSI for 20 minutes, thus allowing the char to be fully permeated with the microbial and mycological tea.
- Activated BioChar has shown an increase of up to 880% in crop yield in volume, and an increase of up to 75% higher nutrition content.
How does one make Abiotic Charcoal?
Pyrolysis VS. Gasification
“Abstract: Biochar produced from biomass pyrolysis is becoming a powerful tool for carbon sequestration and greenhouse gas (GHG) emission reduction.Biochar Crecalcitrance or biochar stability is the decisive property determining it’s carbon sequestration potential. The effect of pyrolysis process parameters on biochar stability is becoming a frontier of biochar study. This review discussed comprehensively how and why Biomass compositions and physicochemical properties and biomass processing conditions such as pyrolysis temperature and reaction residence time affect the stability of biochar. The review found that relative high temperature (400–700 C), long reaction residence time, slow heating rate, high pressure, the presence of some minerals and biomass feedstock of high–lignin content with large particle size are preferable to biochar stability. However, challenges exist to mediate the trade–offs between biochar stability and other potential wins.Strategies were proposed to promote the utilization of biochar as a climate change mitigation tool.” – An overview of the effect of pyrolysis process parameters on biochar stability by Lijian Leng, Huajun Huang
“Thermo-chemical conversion technologies capable of creating biochar include pyrolysis and gasification. Pyrolysis thermally decomposes biomass without the presence of oxygen to create biochar at temperatures starting at 300⁰C. Gasification uses limited oxygen and higher temperatures (500⁰C – 1,500⁰C) (Lehmann et al., 2015). A co-product of biochar production is energy in the form of process heat, liquid fuel, or combustible gases that can be used to supply heat or electricity.
A single laborer can produce 64 tons of biochar per growing season which is incorporated into compost made up of 20% coffee husks, 50% pulp, 20% biochar and 10% top soil. The composting process is still being optimized but currently takes about 8 weeks to finish. 50L/plant of biochar-compost blend is used for new field plantings per tree, of which 2.3 kg is biochar. The blend is deposited in a hole (80 – 100 cm in diameter and ca. 30-40 cm deep) prior to placing the tree. On average the farm spends USD 1,050 per ha for the biochar compost.” – The Potential for Biochar to Improve Sustainability in Coffee Cultivation and Processing: A White Paper
Syngas Biochar Gasifier to produce abiotic Charcoal
Handbook of Biomass Downdraft Gasifier Engine Systems – March 1988
This handbook has been prepared by the Solar Energy Research Institute under the U.S. Department of Energy Solar Technical Information Program. It is intended as a guide to the design, testing, operation, and manufacture of small-scale [less than 200 kW(270 hpJ] gasifiers. A great deal of the information will be useful for all levels of biomass gasification.
Functional Specification for the Biochar
Start by defining the desired qualities and properties of the biochar. These could include:
- Water holding capacity, surface area, pore volume
- Mineralisable and persistent carbon content
- Liming ability, pH, available N, P, K
- Total macro- and micro-nutrient content
- Cation and Anion exchange capacity
- Ability to adsorb heavy metals and other toxic compounds
- Average particle size, bulk and particle density
- Polyaromatic hydrocarbons and dioxin content
See guide “Properties of Fresh and Aged Biochar” for more detail
There are several areas that deserve attention for a solution of what to do with excess carbon, in all of it’s molecular forms
- how to “make” carbon,
- Does carbon need to be cleaned for use,
- how to manage the bi-products of making carbon,
- What is the measurement of carbon capture,
- How long is the carbon captured for
- What is the increase in fruit yield that X amount of carbon will produce
- Carbon Credits can be achieved with what kind of implementation?
- How does a farmer get started with carbon credits?
“According to recent research from Thomas Crowther at ETH Zurich, roughly 3 trillion trees grow on the earth at present and in total, they sequester on the order of 400 Gigatons of carbon dioxide. Crowther and his team figure that enough land exists to be able to plant an additional 1.2 trillion more trees without affecting agricultural production. Were this many trees to be planted, we would succeed in sequestering the better part of the last decade’s worth of carbon emissions.” – Planting 1.2 Trillion Trees Could Cancel Out a Decade of CO2 Emissions, Scientists Find
3,000,000,000,000 trees = 400 Gigatons of Carbon Monoxide (CO) sequestration
“Why trees don’t sequester. Very often, tree planting is recommended to sequester carbon from the atmosphere. This is a misinterpretation of the role of plants in the carbon cycle. Biomass fails to permanently sequester carbon from the atmosphere for several reasons.
- Plants constitute an open system that is in balance with the atmosphere. What is taken up will be released with some time delay. (Figure 4)
- Newly planted biomass will sequester carbon maximally only at the middle of its development to maturation. (Figure 5, solid line). This means that, when you plant a forest for carbon sequestration, the rate of carbon sequestering will increase the first 40-50 years of their growth. After that, the rate will diminish until full growth, when respiration will equal their uptake of carbon.
- At full growth, say 100 – 150 years after the establishment of the forest, the plants have stored carbon maximally (grey field in Figure 5). Any disturbance after this time will release carbon into the air again. So, you cannot harvest the forest, nor should you allow pest, disease or fire.
This is a clearly unsustainable situation. Thus, assuming that increased tree planting will counteract carbon dioxide contamination from fossil fuel burning is, to say the least, a short-sighted solution. Naturally, this is even truer when talking of annual plants, such as most agricultural crops. However, a strategy to increase the dynamic plant cover will increase the amount of the carbon dioxide sequestered from the atmosphere. Some such strategies will be discussed below.
Due to its porosity and thus its large internal area, up to 1500 m2/g 17, charcoal has an excellent capacity to adsorb nutrients and organic material, and hence also works as a very good habitat and growth area for soil micro-organisms. Therefore, in any poor soil, such as excessively sandy, clayey or leaky soils, the addition of charcoal is a good way to improve it. The charcoal works as a ‘sponge’ for the nutrients, which due to the increased microbial biomass are accessible for the plants growing nearby. (Plants ‘buy’ nutrients from micro-organisms with sugars released from their roots). Charcoal also exerts significant effects on the decomposition of added litter. The increased amount of microbial biomass has also a positive effect of the growth of earthworm populations (which feed on micro-organisms), something that will further augment the productivity of the soil18.
http://www.holon.se/folke/carbon/Terra%20pretav1_0.pdf
Glossary:
- A Retort is an airtight vessel in which substances are externally heated, usually producing gases to be collected in a collection vessel, or for further processes.
- Batch Pyrolysers are simple low-cost devices that are filled with biomass, run to completion and then emptied.
- Basic batch stoves, retorts and kilns are often used for small-scale manufacture of biochar, and also for larger scale production of fuel- or process-charcoal (eg for reducing metals).
- Continuous Pyrolysers are devices where biomass is fed into one end while biochar is continuously discharged from the other.
Continuous devices are more complex and expensive, but can provide:- more production from a given amount of equipment and labor
- more control over the process conditions of the biochar
- lower emissions
- Reactor (or more specifically a chemical reactor) is a vessel designed to contain and control (chemical) reactions.
- A Pyrolyser is a reactor designed for thermal decomposition of biomass in a limited oxygen environment (= pyrolysis).
- A Gasifier is a reactor in which air is intentionally injected into the feedstock. Part of the feedstock is burned to produce a relatively clean pyrogas. A gasifier usually operates at a higher temperature than a pyrolyser.
- A Stove is an enclosed space in which fuel is burned to provide heating, either to heat the stove itself and the space in which it is situated, or to heat items placed on the stove.
- A Kiln is a kind of oven, a thermally insulated chamber,that produces temperatures sufficient to complete some process, such as drying, or chemical change. A kiln may be internally or externally heated.
- Pyrogas (or Pyrolysis gas): The gas and aerosols from pyrolysis or gasification comprising primarily combustible gases CO, H2and CH4along with CO2, steam and N2; also known as wood gas and syngas.
- Primary Air (PA): In pyrolysis PA refers to air supplied to the fuel bed, needed to partially combust the material resulting in emission of combustible vapoursand gases.If pyrolysis is sustained by external heat, PA provides a fraction of the air required for first stage combustion of emitted gases.
- Secondary Air (SA): (and in some instances tertiary air) refers to additional air injected to the combustion zone to complete combustion of the fuel gases.
- Materials Handling: This refers to the equipment that moves the biomass to the pyrolyserand moves the biochar from the pyrolyser.
- Materials Preparation Equipment: This includes machinery that reduces the size (e.g. grinders), compacts the biomass into pellets or briquettes, dries the biomass, or mixes ingredients (such as biomass and minerals) together.